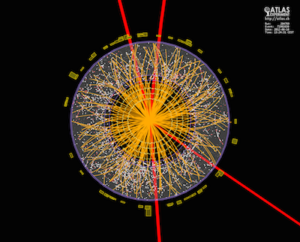
Elementary particle physics is the study of the smallest known components of matter and their mutual interactions. The mathematical language for describing such particles is that of quantum field theory, the result of merging the theory of special relativity with quantum mechanics. How to fully merge general relativity and quantum mechanics
is still an open issue.
For many decades we have had a working quantum field theory, the “Standard Model”, providing a coherent picture of the world as built out of basic building blocks (quarks and leptons) interacting through the exchange of other particles called vector bosons. The last ingredient required for this theory to be complete, the Higgs boson, was discovered in 2012 at the Large Hadron Collider (LHC) at CERN.
Among the quarks there are those that make up the protons and neutrons found in the atomic nucleus. They are called up and down quarks. Among the leptons we find the electron that, together with the nucleus, makes up all the atoms in the periodic table. Finally, the prime example of a vektor boson is the particle of light itself — the photon — responsible for the description of all electromagnetic interactions. There are other particles of each type, most of which are unstable and not found in ordinary matter, describing more exotic phenomena.
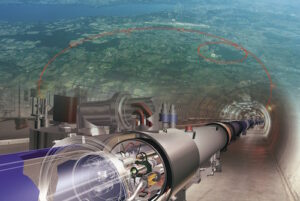
The experimental tools that have been used to test the Standard Model comprise first of all of particle colliders, such as the LHC at CERN, near Geneva, where the energy of the colliding particles in the beam is converted into new heavy particles according to Einstein’s well known relation between mass and energy. There have also been a number of highly valuable non-collider experiments that helped elucidating e.g. the nature of neutrinos (types of lepton).
Our group is involved in theoretical attempts at formulating and testing even more complete and overarching theories that would include phenomena beyond the Standard Model. There are many reasons to believe that such a theory can be formulated, perhaps the most compelling one being the existence of dark matter, a type of matter not present in the Standard Model, interacting very weakly with ordinary matter but whose presence can be inferred by its gravitational effects. Many models of physics beyond the Standard Model involve particles that could be candidate constituents of such dark matter component of the universe. These particles could also be produced in collider experiments and their properties studied in a controlled environment. Additional motivations include finding an explanation for the mass of the Higgs boson and for why the universe is made of matter and not anti-matter.
The theoretical activity is divided into a model building phase, where new ideas are implemented in a model, typically involving new particles or new interactions, and a simulation phase, where the model is used to predict some experimental outcome and cross checks with the already available experimental data are performed.
The first phase relies for guidance on the notion of symmetry, namely on the existence of transformations among the quantities of the theory that leave the theory unchanged. Such concept have been at the heart of many previously successful constructions, such as the theory of special relativity, that can be based upon the postulate of invariance under space-time transformation known as Poincarè transformations, or electrodynamics, based on so called “gauge” invariance.
At the phenomenological level, any new symmetry (if realized in nature) predicts the existence of a new set of particles in addition to those of the Standard Model and also dictates many properties of their interactions. Such interactions can then be simulated on a computer and the outcomes compared with experiment. It is a truly amazing accomplishment of the Standard Model that it has so far passed all the collider-based tests, but the hunt continues!