You can read more about the projects and the research conducted on Chalmers Research, or click the direct links in the items below.
Extending the reach of uncertainty quantification in nuclear theory
by Isak Svensson
ISBN 978-91-7905-823-4 (2023)
The theory of the strong interaction—quantum chromodynamics (QCD)—is unsuited to practical calculations of nuclear observables and approximate models for nuclear interaction potentials are required. In contrast to phenomenological models, chiral effective field theories (χEFTs) of QCD grant a handle on the theoretical uncertainty arising from the truncation of the chiral expansion. Uncertainties in χEFT are preferably quantified using Bayesian inference, but quantifying reliable posterior predictive distributions for nuclear observables presents several challenges. First, χEFT is parametrized by unknown low-energy constants (LECs) whose values must be inferred from low-energy data of nuclear structure and reaction observables. There are 31 LECs at fourth order in Weinberg power counting, leading to a high-dimensional inference problem which I approach by developing an advanced sampling protocol using Hamiltonian Monte Carlo (HMC). This allows me to quantify LEC posteriors up to and including fourth chiral order. Second, the χEFT truncation error is correlated across independent variables such as scattering energies and angles; I model correlations using a Gaussian process. Third, the computational cost of computing few- and many-nucleon observables typically precludes their direct use in Bayesian parameter estimation as each observable must be computed in excess of 100,000 times during HMC sampling. The one exception is nucleon-nucleon scattering observables, but even these incur a substantial computational cost in the present applications. I sidestep such issues using eigenvector-continuation emulators, which accurately mimic exact calculations while dramatically reducing the computational cost. Equipped with Bayesian posteriors for the LECs, and a model for the truncation error, I explore the predictive ability of χEFT, presenting the results as the probability distributions they always were.
Approximating the Three-Nucleon Continuum
– Solving the Faddeev equations for statistical inference of chiral forces –
by Sean Miller
ISBN 978-91-7905-721-3 (2022)
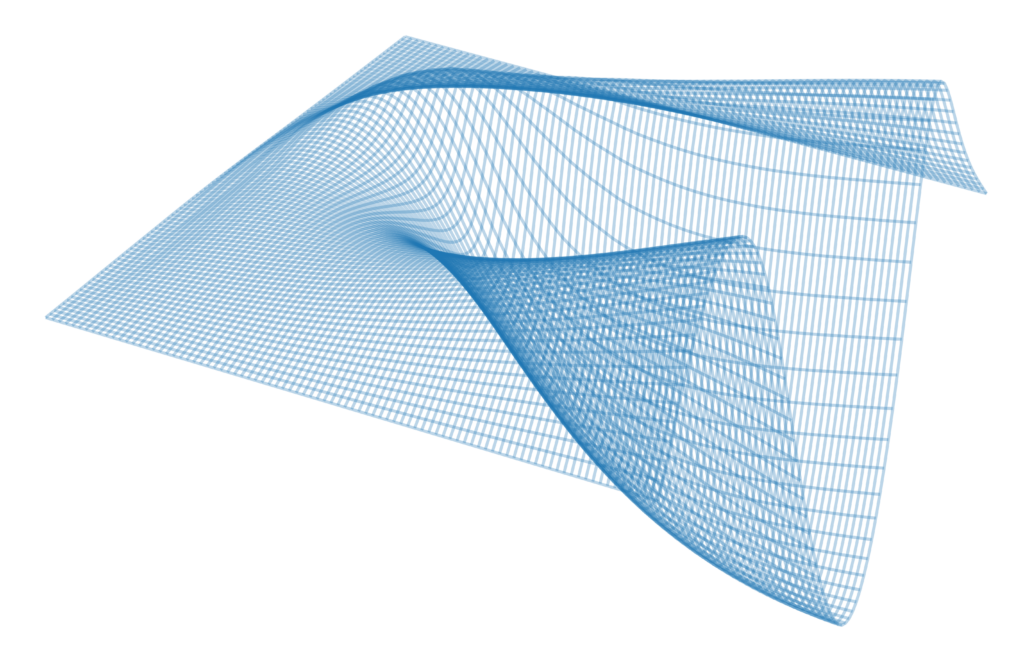
Three-nucleon forces (3NFs) are necessary to accurately describe the properties of atomic nuclei. These forces arise naturally together with two-nucleon forces (2NFs) when constructing nuclear interactions using chiral effective field theories (χEFTs) of quantum chromodynamics. Unlike phenomenological nuclear interaction models, χEFT promises a handle on the theoretical uncertainty in our description of the nuclear interaction. Recently, methods from Bayesian statistics have emerged to quantify this theoretical truncation error in physical predictions based on chiral interactions. Alongside quantifying the truncation error, the low-energy constants (LECs) of the chiral interactions must be inferred using selected experimental data. In this regard, the abundant sets of experimentally measured nucleon-nucleon (NN) and nucleon-deuteron (Nd) scattering cross sections serve as natural starting points to condition such inferences on. Unfortunately, the high computational cost incurred when solving the Faddeev equations for Nd scattering has thus far hampered Bayesian parameter estimation of LECs from such data. In this thesis, I present the results from a two-part systematic investigation of the wave-packet continuum discretisation (WPCD) method for reliably approximating two- and three-nucleon (NNN) scattering states with an aim towards a quantitative Bayesian analysis in the NNN continuum. In the first part, I explore the possibilities of using graphics processing units to utilise the inherent parallelism of the WPCD method, focusing on solving the Lippmann-Schwinger equation. In the second part, I use the WPCD method to solve the Faddeev equations for Nd scattering and analyse the reliability of the approximations of the WPCD method. This allows me to quantify the posterior predictive distributions for a range of low-energy neutron-deuteron cross sections conditioned on NN scattering data and NN interactions up to fourth order in χEFT.
JupiterNCSM: A Pantheon of Nuclear Physics
—an implementation of three-nucleon forces in the no-core shell model—
by Tor Djärv
ISBN 978-91-7905-552-3 (2021)
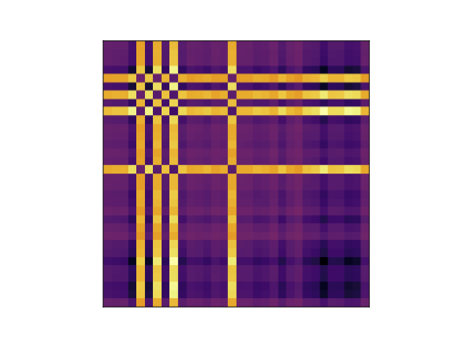
It is well established that three-nucleon forces (3NFs) are necessary for achieving realistic and accurate descriptions of atomic nuclei. In particular, such forces arise naturally when using chiral effective field theories (χEFT). However, due to the huge computational complexity associated with the inclusion of 3NFs in many-body methods they are often approximated or neglected completely. In this thesis, three different methods to include the physics of 3NFs in the ab initio no-core shell-model(NCSM) have been implemented and tested. In the first method, we approximate the 3NFs as effective two-body operators by exploiting Wick’s theorem to normal order the 3NF relative a harmonic-oscillator Slater determinant reference state and discarding the remaining three-body term. We explored the performance of this single-reference normal-ordered two-body approximation on the ground-state energies of the two smallest closed-core nuclei, 4He and 16O, in particular focusing on consequences of the breaking of translational symmetry. The second approach is a full implementation of 3NFs in a new NCSM code, named JupiterNCSM, that we provide as an open-source research software. We have validated and benchmarked JupiterNCSM against other codes and we have specifically used it to investigate theeffects of different 3NFs on light p-shell nuclei 6He and 6Li. Finally, we implement the eigenvector continuation (EVC) method to emulate the response of ground-state energies of the aforementioned A = 6 nuclei to variations in the low-energy constants of χEFT that parametrize the 3NFs. In this approach, the full Hamiltonian is projected onto a small subspace that is constructed from a few selected eigenvectors. These training vectors are computed with JupiterNCSM in a large model space for a small set of parameter values. This thesis provides the first EVC-based emulation of nuclei computed with a Slater-determinant basis. After the training phase, we find that EVC predictions offer a very high accuracy and more than seven orders
of magnitude computational speedup. As a result we are able to perform rigorous statistical inferences to explore the effects of 3NFs in nuclear many-body systems.
Unveiling the nature of dark matter with direct detection experiments
by Vanessa Zema
ISBN 978-91-7905-354-3 (2020)
The desire of discovery is an anthropic need which characterises and connects the human being over the eras. In particular, observing the sky is an instinctive drive exerted by the curiosity of the mysteries which it retains. At the present time, the tremendous advances in the exploration of space have opened even more challenges than back in the days. One of the most urgent question is unveiling the nature of dark matter (DM). As stated by Neta A. Bahcall (Professor at Princeton University), “Cosmology has revealed an amazing universe, filled with a “dark sector” that composes 95% of the energy density of our cosmos […]” (Dark matter universe, PNAS, 2015). About one-third of this dark sector is associated to an invisible and still undetected form of matter, the so-called dark mat- ter, whose gravitational effect manifests at all cosmological scales. Both theoretical and experimental observations based on ordinary gravity reinforced the evidences for the existence of DM, since its first appearance in the pioneering calculations of F. Zwicky (1933). This PhD project explores the hypothesis that DM is made of new particles beyond the standard model. More specifically, it focuses on those DM particles which are trapped into the galactic gravitational field and populate the galactic halo. If DM interacts with ordinary particles, extremely sensitive detectors operating in very low-background envi- ronments, are expected to detect galactic DM particles scattering off their target material. This widely employed experimental technique is known as DM direct detection and it is the focus of my studies, where I consider the further hypothesis that DM interacts with atomic nuclei. The research I conducted during my PhD program consists of two main parts: the first part focused on purely phenomenology aspects of the DM direct detection (namely on the DM annual modulation treated using a non-relativistic effective theory and on the scattering of spin-1 DM particles off polarised nuclei) and the second one is more closely connected to experimental applications. The latter has been strongly stimulated by my collaboration with the two DM direct detection experiments CRESST and COSINUS. For CRESST, I compute the DM-nucleus cross-section for the conventional spin-dependent interactions, used to analyse the data collected with a prototype Li-based detector module, and I derive some prospects for a time dependent analysis of CRESST-III data, using a sta- tistical frequentist approach based on Monte Carlo simulations. For COSINUS, I provide a significant extension of the pulse shape model currently used by CRESST and COSINUS in order to explain experimental observations related to the COSINUS detector response. Finally, I contribute to ongoing studies on the phonon propagation in NaI crystals based on solid state physics. This PhD thesis has been oriented to fill the gap between theoretical and experimental efforts in the DM field. This approach has facilitated the exchange of expertise, has driven the trend of my research and has stimulated the development of the ideas and methods described in this PhD thesis.
The data perspective on chiral effective field theory
by Boris Carlson
ISBN 978-91-7597-530-6 (2017)
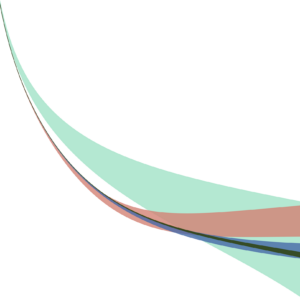
The scientific method implies a dynamical relationship between experiment and theory. Indeed, experimental results are understood through theories, which themselves are of less value until confronted with experiment. In this thesis I study this relationship by quantifying two key properties of theories: theoretical uncertainties and predictive power. Specifically I investigate chiral effective field theory and the precision and accuracy by which it reproduces and predicts low-energy nuclear observables. I estimate both statistical and systematic uncertainties. The conclusion is that the latter, which in my approximation originates from omitted higher-order terms in the chiral expansion, are much larger than the former. In relation to this, I investigate the order-by-order convergence up to fourth order in the chiral expansion. I find that predictions generally improve with increasing order, while the additional low-energy constants (LECs) of the interaction makes it more difficult to fully constrain the theory. Furthermore, in order to accurately reproduce properties of heavier nuclei I see indications that it is necessary to include selected experimental data from such systems directly in the fitting of the interaction. In order to perform these studies I have developed accurate and efficient methods as well as computer codes for the calculation of observables. In particular, the application of automatic differentiation for derivative calculations is shown to be crucial for the minimization procedure. These developments open up new avenues for future studies. For example, it is now possible to do extensive sensitivity analyses of the experimental data and the model; to investigate the power counting from a data perspective; and incorporate more experimental data in the fitting procedure.of magnitude computational speedup. As a result we are able to perform rigorous statistical inferences to explore the effects of 3NFs in nuclear many-body systems.
Cluster Effective Field Theory
by Emil Ryberg
ISBN 978-91-7597-492-7 (2016)
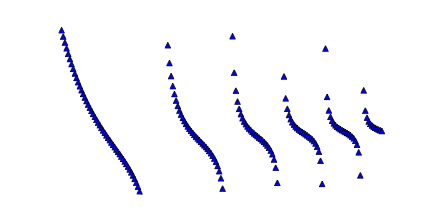
Halo nuclei are loosely bound systems consisting of a core plus valence nucleon(s). In so called Halo, or Cluster, effective field theory, the core of the halo nucleus is treated as an effective degree-of-freedom without internal structure. As such, Cluster effective field theory is a low-energy model, appropriate for the typical momentum scales of halo physics. The advantages of using effective field theory are the systematic way of improving results, by including higher orders in the momentum expansion, and the rigorous error estimates that are available at each order. In this thesis we present a formalism for treating one-proton and two-neutron halo nuclei in effective field theory, with an emphasis on charge radii, astrophysical S-factors, and the renormalization of three-body states. We also discuss a new power-counting scheme for heavy-core systems and introduce finite-size contributions. For one-proton halo nuclei we derive formalism for S- and P-wave systems, which we exemplify by studying the one-proton halo states 17F* and 8B, respectively. Of particular significance are: (i) our calculation of the radiative capture cross section of 16O(p,gamma)17F* to fifth order in the S-wave system and (ii) our derivation of a leading-order correlation between the charge radius of 8B and the threshold S-factor of 7Be(p,gamma)8B for the P-wave system. Our alternative power counting for halo nuclei with a heavy core leads to a new organizational principle that demotes the naive leading-order contributions to the charge radius for neutron halos. Additionally, in this new power counting we include the finite-size effects of the constituents explicitly into the field theory and derive how their finite sizes contribute to the charge radius of S- and P-wave one-neutron and one-proton halo states. For two-neutron halo systems we derive the field-theory integral equations to study both bound and resonant states. We apply the formalism to the 0+ channel of 6He. In this three-body field theory we include both the 3/2- and the 1/2- channels of the alpha-n subsystem, together with the 0+ channel of the n-n part. Furthermore, we include the relevant three-body interactions and analyze, in particular, the renormalization of the system.
Bridging scales in nuclear physics
by Daniel Sääf
ISBN 978-91-7597-408-8 (2016)
In this thesis we present the ab initio no-core shell model (NCSM) and use this framework to study light atomic nuclei with realistic nucleon-nucleon interactions. In particular, we present results for radii and ground-state energies of systems with up to twelve nucleons. Since the NCSM uses a finite harmonic oscillator basis, we need to apply corrections to compute basis-independent results. The derivation, application, and analysis of such corrections constitute important results that are presented in this thesis. Furthermore, we compute three-body overlap functions from microscopic wave functions obtained in the NCSM in order to study the onset of clusterization in many-body systems. In particular, we study the Borromean two-neutron halo state in 6He by computing the overlap function < 6He(0+)|4He(0+) + n + n >. We can thereby demonstrate that the clusterization is driven by the Pauli principle. Finally, we develop state-of-the-art computational tools to efficiently extract one- and two-body transition densities from microscopic wave functions. These quantities are important properties of many-body systems and are keys to compute structural observables. In this work we study the core-swelling effect in 6He by computing the average distance between nucleons.